Planck Satellite
Planck, a European Space Agency satellite, launched on May 14, 2009, that measured the cosmic microwave background (CMB), the residual radiation left over from the big bang, at a much greater sensitivity and resolution than was provided by the U.S. Wilkinson Microwave Anisotropy Probe (WMAP). It was named in honour of German physicist Max Planck, a pioneer in quantum physics and in the theory of blackbody radiation. It was launched on an Ariane 5 rocket that also carried Herschel, an infrared space telescope.
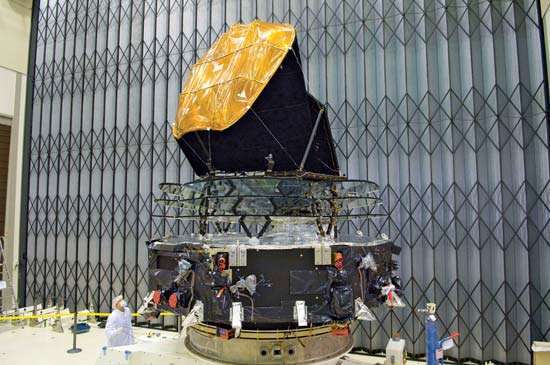
- The mission had a wide variety of scientific aims, including:
- high resolution detections of both the total intensity and polarization of primordial CMB anisotropies,
- creation of a catalogue of galaxy clusters through the Sunyaev–Zel'dovich effect,
- observations of the gravitational lensing of the CMB, as well as the integrated Sachs–Wolfe effect,
- observations of bright extragalactic radio (active galactic nuclei) and infrared (dusty galaxy) sources,
- observations of the Milky Way, including the interstellar medium, distributed synchrotron emission and measurements of the Galactic magnetic field, and
- studies of the Solar System, including planets, asteroids, comets and the zodiacal light.
Planck had a higher resolution and sensitivity than WMAP, allowing it to probe the power spectrum of the CMB to much smaller scales (×3). It also observed in nine frequency bands rather than WMAP's five, with the goal of improving the astrophysical foreground models.
It is expected that most Planck measurements will be limited by how well foregrounds can be subtracted, rather than by the detector performance or length of the mission, a particularly important factor for the polarization measurements. The dominant foreground radiation depends on frequency, but could include synchrotron radiation from the Milky Way at low frequencies, and dust at high frequencies.
Like WMAP, Planck was positioned near the second Lagrangian point (L2), a gravitational balance point between Earth and the Sun and 1.5 million km (0.9 million miles) opposite the Sun from Earth. The spacecraft moved in a controlled Lissajous pattern around L2 rather than “hovering” there. This isolated the spacecraft from radio emissions from Earth and the Moon without having to place it on a more distant trajectory that would complicate tracking. The spacecraft spun once per minute and shifted its rotational axis every 15 minutes to shield itself from the Sun. Five complete scans of the sky were made during the mission, which ended in 2013.
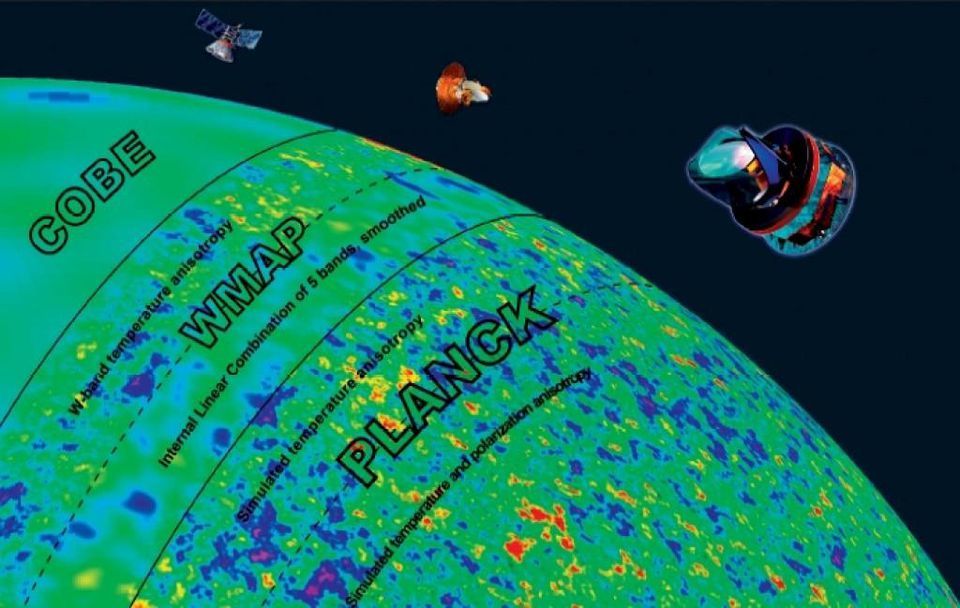
It's been more than 50 years since humanity discovered a uniform bath of low-energy, microwave radiation originating from all regions of the sky. It doesn't come from the Earth, the Sun, or even the galaxy; it originates beyond every star or galaxy we've ever observed. While it's discoverers didn't know what it initially was, a group of nearby physicists were in the midst of designing an experiment to look for that exact signature: the theoretical leftover glow from the Big Bang.
Initially known as the primeval fireball, we now call in the cosmic microwave background (CMB), having measured its properties exquisitely. The most advanced observatory to ever measure its properties is the European Space Agency's Planck satellite, launched in 2009. The satellite took its full suite of data over many years, and the scientists working on it have just completed and released their final analysis. Here's how it's changed our view of the Universe forever.
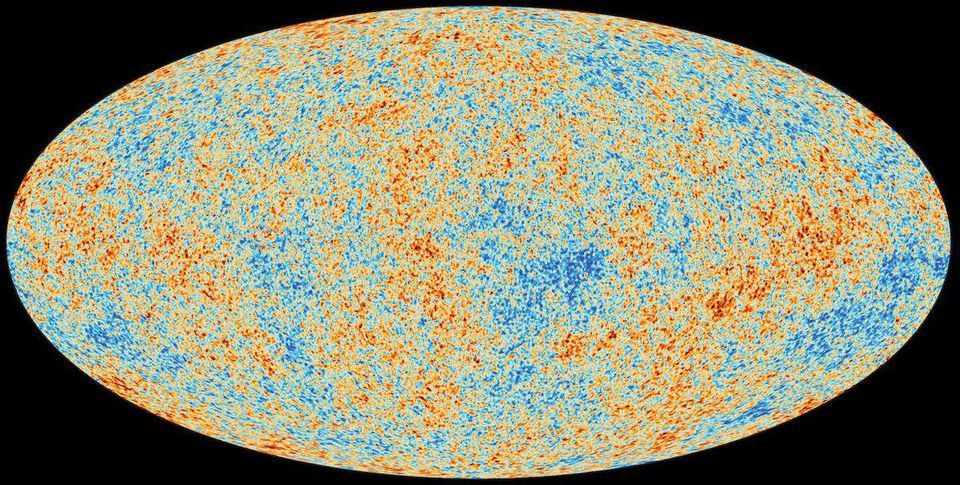
The leftover glow from the Big Bang, the CMB, isn't uniform, but has tiny imperfections and temperature fluctuations on the scale of a few hundred microkelvin. While this plays a big role at late times, after gravitational growth, it's important to remember that the early Universe, and the large-scale Universe today, is only non-uniform at a level that's less than 0.01%.
This baby picture of the Universe, whose light was emitted when the Universe was only 380,000 years old, is far more exquisite than any that came before. In the early 1990s, the COBE satellite gave us the first precision, all-sky map of the cosmic microwave background, down to a resolution of about 7 degrees. About a decade ago, WMAP managed to get that down to about half-a-degree resolution.
But Planck? Planck is so sensitive that the limits to what it can see aren’t set by instruments, which can measure down to 0.07° or so, but by the fundamental astrophysics of the Universe itself! In other words, it will be impossible to ever take better pictures of this stage of the Universe than Planck has already taken. Additional resolution will not get you better information about our cosmos.
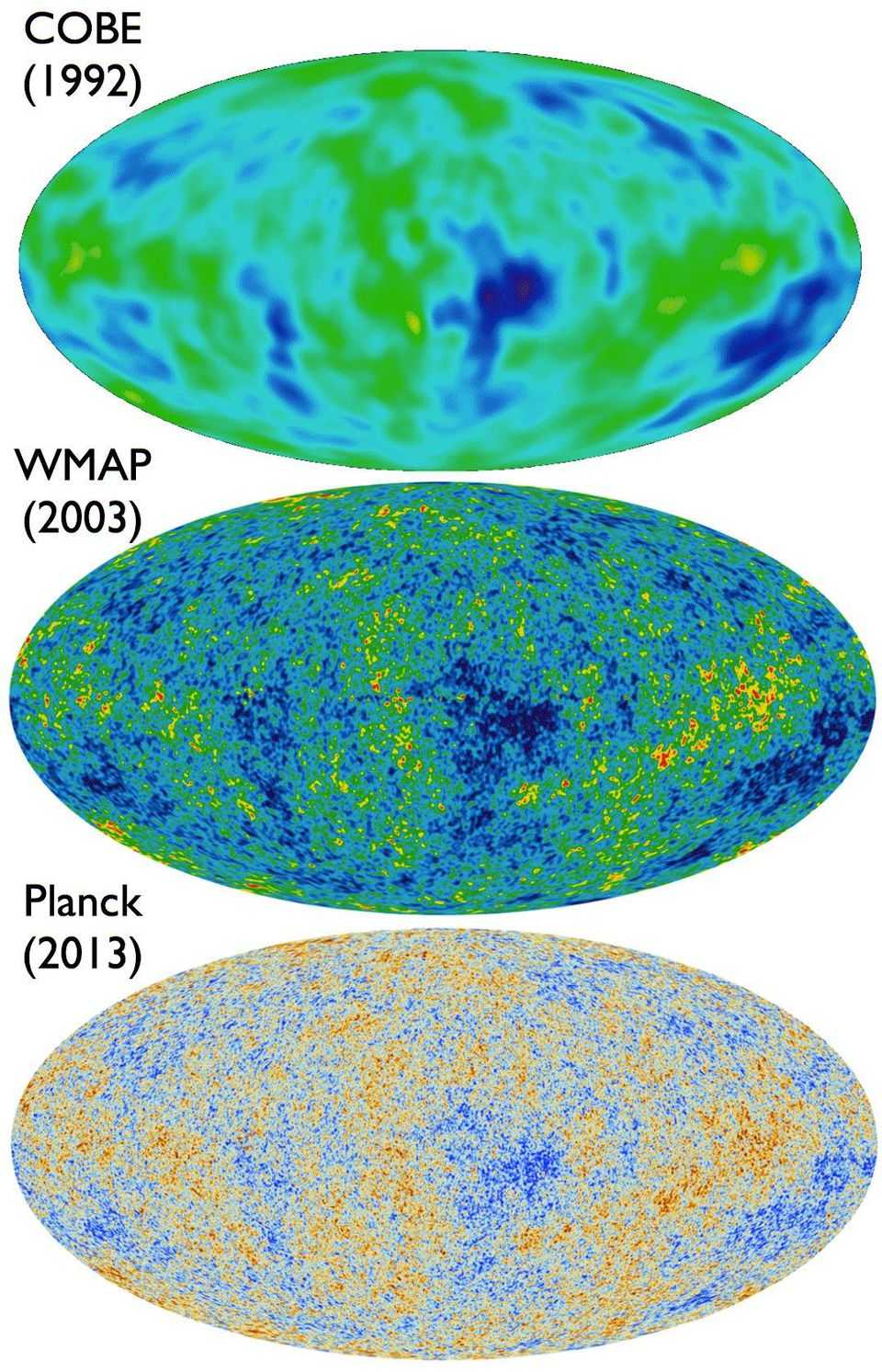
Planck has also measured this radiation and its fluctuations in more frequency bands (nine in total) than any satellite that came before. COBE had four (only three were useful), and WMAP had five. COBE could measure temperature fluctuations that were approximately 70 microkelvin (µK) in magnitude; Planck can get down to precisions of around ~5 µK or better.
The high resolution, the capabilities of measuring the polarization of this light, and the multiple frequency bands have enabled us to understand, measure, and subtract out the effects of dust in our galaxy better than ever before as well. If you want to understand the Big Bang's leftover glow, you have to understand to equal-or-better precision the effects that could contaminate that signal. Before we extract any cosmological information, that step needed to happen.
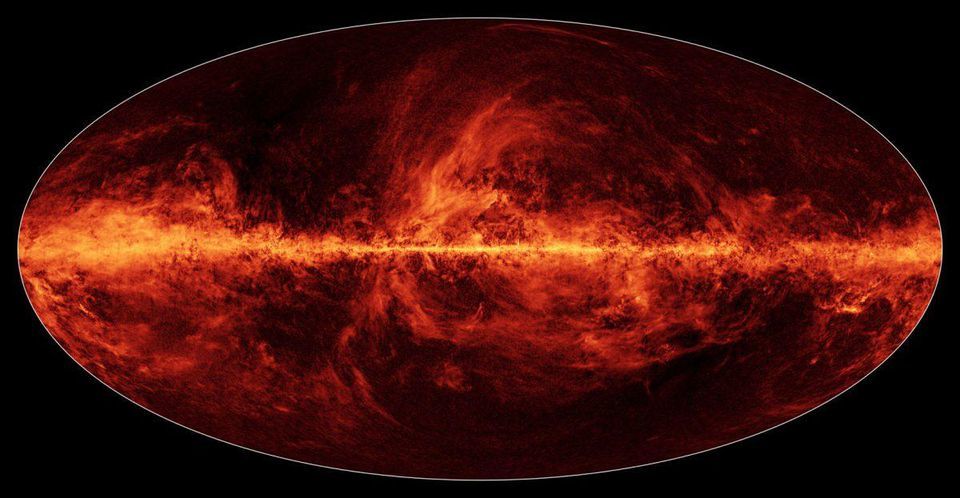
Once you have the full signal from the early Universe, you can then analyze it to extract all the information possible. This means using the temperature fluctuations on large, intermediate, and small scales to figure out things like:
- how much normal matter, dark matter, and dark energy are in the Universe,
- what the initial distribution and spectrum of density fluctuations were,
- and what the shape/curvature of the Universe is.
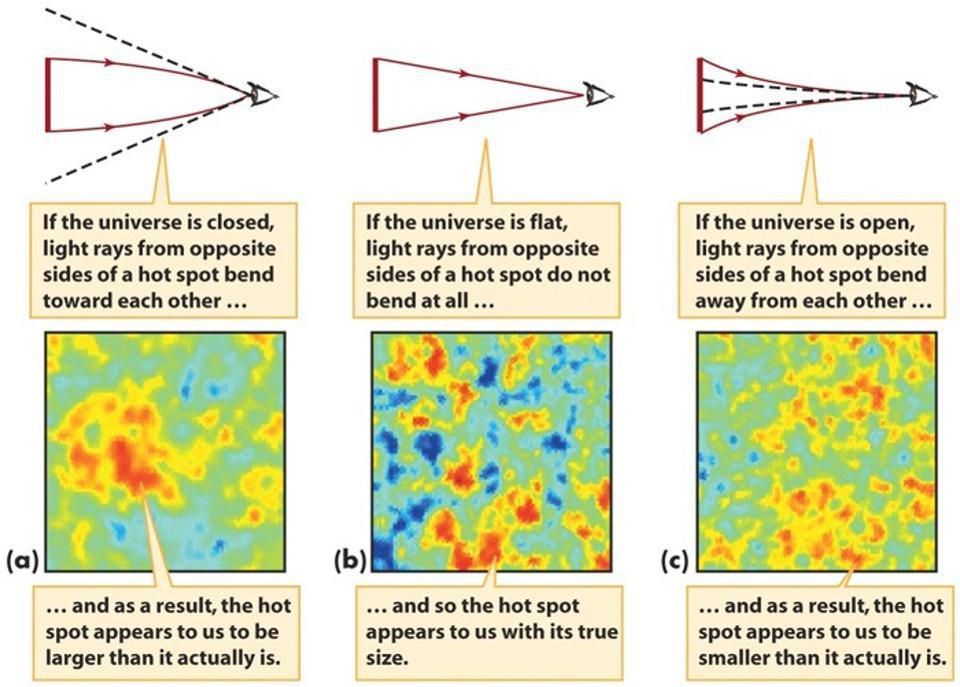
What happens on different scales are all independent of one another, but are highly dependent on what the Universe is made of. We can also look at a variety of polarization signatures of this radiation, and learn even more, such as:
- when the Universe became reionized (and, therefore, when star formation reached a certain threshold),
- whether there are fluctuations on scales larger than the horizon,
- whether we can see the effects of gravitational waves,
- what the number and temperature of neutrinos were back then,
and much more. While the temperature of the CMB is still 2.725 K, the same value it's been known to have for decades, many other things have changed. With all that, here's how our view of the Universe has forever been changed by Planck.
The Universe has more matter and is expanding more slowly than we previously thought. Before Planck, we thought the Universe was about 26% matter and 74% dark energy, with an expansion rate (in units of km/s/Mpc) in the low-70s.
he Universe is 31.5% matter (where 4.9% is normal matter and the rest is dark matter), 68.5% dark energy, with a Hubble expansion rate today of 67.4 km/s/Mpc. That latter figure has such small uncertainties on it (~1%) that it's in tension with measurements from the cosmic distance ladder, which indicate a rate closer to 73 km/s/Mpc. This last point is probably the biggest remaining controversy surrounding our modern view of the Universe.
We also have the best-ever confirmation that the density fluctuations align perfectly with what the theory of cosmic inflation predicts. The simplest inflation models predict that the fluctuations the Universe was born with would be almost, but not quite, the same on all scales, with slightly larger fluctuations on large scales than small ones.
For Planck, this means one of the quantities it can derive, n_s, should be almost equal to 1, but just a little less. Planck's measurements are the most precise ever, and confirm inflation's spectacularly: n_s = 0.965, with an uncertainty of less than 0.05%.
There's also the question of whether dark energy is truly a cosmological constant or not, which is extremely sensitive to both the CMB and the data from the ultra-distant Universe, such as Type Ia supernovae. If dark energy is a perfect cosmological constant, its equation of state, given by the parameter w, should equal -1 exactly.
We find that w = -1.03, with an uncertainty of 0.03. There is no evidence for anything else, meaning that both the Big Crunch and the Big Rip scenarios are not favored by the data.
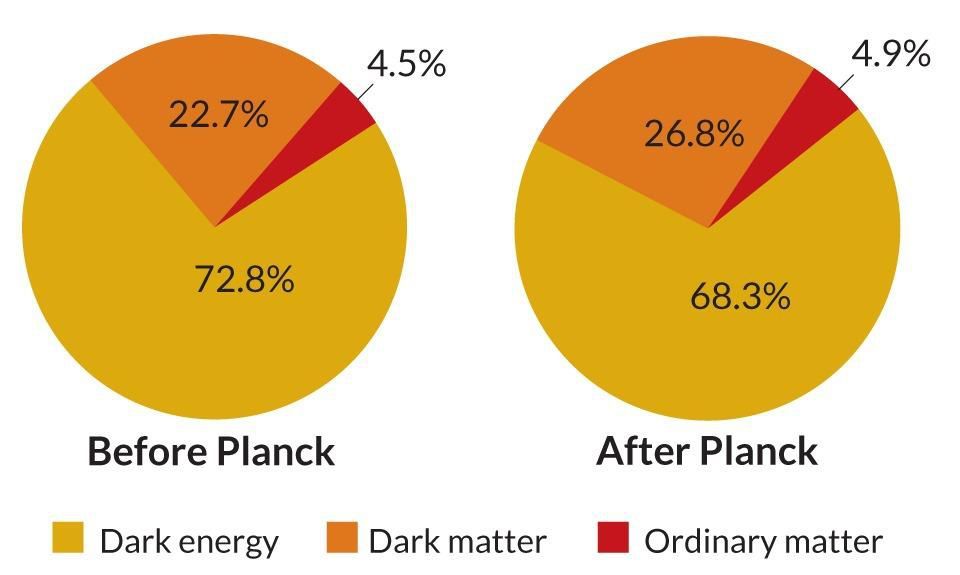
Most importantly, a spectacular agreement to a never-before-achieved precision now exists between the CMB we observe and the theoretical predictions of a Universe with 5% normal matter, 27% dark matter, and 68% dark energy. There might be wiggle room of 1-2% in some of those numbers, but a Universe without dark matter and dark energy, both, in great abundance, is a no-go in the face of these observations. They're real, they're necessary, and their predictions match the full suite of data perfectly.
Inflation, neutrino physics, and the Big Bang have additional pieces confirming them, while alternatives and specific variants are better constrained. Most definitively, the Planck collaboration states, "We find no compelling evidence for extensions to the base-ΛCDM model." At last, we can state, with extraordinary confidence, what the Universe is made of.
A common service module (SVM) was designed and built by Thales Alenia Space in its Turin plant, for both the Herschel Space Observatory and Planck missions, combined into one single program.
The overall cost is estimated to be €700 million for the Planck and €1,100 million for the Herschel mission. Both figures include their mission's spacecraft and payload, (shared) launch and mission expenses, and science operations.
Structurally, the Herschel and Planck SVMs are very similar. Both SVMs are octagonal in shape and each panel is dedicated to accommodate a designated set of warm units, while taking into account the dissipation requirements of the different warm units, of the instruments, as well as the spacecraft. On both spacecraft, a common design was used for the avionics, attitude control and measurement (ACMS), command and data management (CDMS), power, and tracking, telemetry and command (TT&C) subsystems. All units on the SVM are redundant.
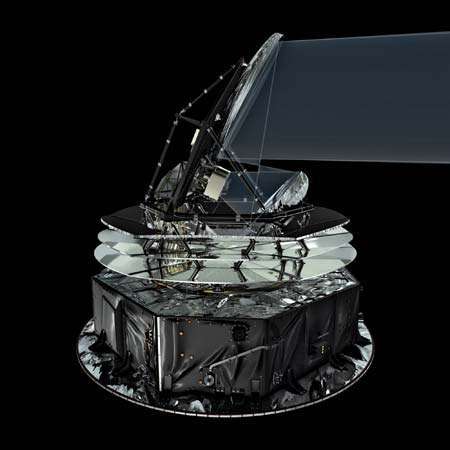
Planck’s instruments covered radio emissions from 30 to 857 gigahertz and measured temperature fluctuations in the CMB with a precision of about 2 parts per million at an angular resolution of about 10 minutes of arc. These temperature fluctuations in turn indicate density fluctuations from which the first galaxies formed. The high angular resolution and the polarization of the instruments allowed Planck to measure the Sunyaev-Zeldovich effect, a distortion of the CMB caused by galaxy clusters, and to observe gravitational lensing in the CMB.
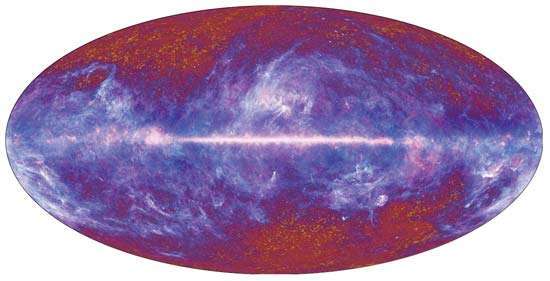







REFERENCES
Encyclopædia Britannica. Available in: https://www.britannica.com/topic/Planck. Acces in: 20/12/2018.
Forbes. Available in: https://www.forbes.com/sites/startswithabang/2018/07/19/how-the-planck-satellite-changed-our-view-of-the-universe/#10814ec77ad2. Acces in: 20/12/2018.
Wikipedia. Available in: https://en.wikipedia.org/wiki/Planck_(spacecraft). Acces in: 20/12/2018.
BBC. Available in: https://www.bbc.com/news/science-environment-21866464. Acces in: 20/12/2018.
University of Cambridge. Available in: http://www.ctc.cam.ac.uk/news/130322_newsitem.php. Acces in: 20/12/2018.
Nasa. Available in: https://www.nasa.gov/jpl/planck/pia18913. Acces in: 20/12/2018.
Nasa. Available in: https://www.nasa.gov/jpl/planck/pia18912. Acces in: 20/12/2018.
Nasa. Available in: https://www.nasa.gov/jpl/pia19330/astronomers-dig-up-treasure-trove-of-galaxy-clusters. Acces in: 20/12/2018.
European Space Agency. Available in: https://www.esa.int/Our_Activities/Space_Science/Planck. Acces in: 20/12/2018.
European Space Agency. Available in: https://www.cosmos.esa.int/web/planck. Acces in: 20/12/2018.
Cardiff University. Available in: http://planck.cf.ac.uk/mission. Acces in: 20/12/2018.
0 comments
Sign in or create a free account